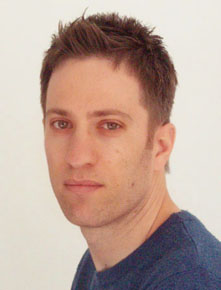 |
Research Overview
The current cosmological model successfully accounts for the global
features of the universe, such as the spatial distribution of mass
in the universe, but apparently fails below cluster scales. The
results of current simulations still disagree with observational
data, particularly so for the cores of clusters. The discrepancy is
apparent when comparing observed and predicted scaling relations,
such as the luminosity-temperature relation, entropy-temperature
relation, and the mass-temperature relation. Other intracluster (IC)
gas properties, such as higher chemical elements (hereafter
metallicity) distribution, entropy and - most notably - the central
mass profile, especially the prediction of a cusp in the DM density
in the inner core, are also in conflict with observational results.
Unexplained features on galactic scales include high predicted
numbers of small satellite systems around giant galactic halos, and
low angular momentum and the consequent small size of galactic
disks. Moreover, both theoretical models and numerical results
significantly overpredict the observed stellar mass density at early
times.
I argue that these differences can be a consequence of incorrect
modeling of the star formation history, and lack of essential
physical processes in semi-analytical models and simulations. Thus,
in the current research I will test the effects of implementing the
essential physical processes that occur in the cluster galaxies and
in IC gas, combined with higher and more realistic star formation
rates at early times, on the properties of clusters of galaxies and
their basic statistical scaling relations.
My basic idea is to model the galaxies in the cluster as basic
constructs (galcons) with an extended distribution. Then, by using
semi-analytic models for the physical phenomena in those galaxies, I
will be able to describe the global mass and energy feedback from
galaxies to the IC gas, instead of calculating the feedback from
each individual star. This simplification will substantially reduce
the required computational resources without affecting the total
mass distribution, therefore increasing the simulation spatial
resolution. The main benefit will be the attainment of a higher
level of spatial resolution and a more detailed and complete
description of the interactions between the galaxies, DM and gas.
Furthermore, the necessary physical processes can be easily
implemented in the code using semi-analytic models, and it will also
be possible to generate relatively long episodes of star formation
in these galaxies, as seen in recent observations.
All these modifications will be implemented in a powerful
cosmological hydrodynamical simulation code.
Physical processes that
will be studied in this research, such as outflows of energy and
mass from stellar explosions, large amount of gas stripped from the
cluster galaxies, dynamical friction between the galaxies, gas and
DM, will strongly affect the properties of IC gas. Previous studies which ignored these processes, obviously enhanced the
discrepancy with observations. Furthermore, different levels of
activity, combination, and duration of these processes will shape IC
gas in different ways. Therefore, I hope to be able to draw some
conclusions about the strength and effectiveness of these processes
on cluster properties.
It is anticipated that our planned simulation and comprehensive
analysis work will lead to an improved description of the physical
phenomena in galaxies and clusters, and to better insight on the
history of star formation in galaxies. This will enhance our
understanding of the large scale structure of the universe and the
detailed properties of the largest (physically bound) systems –
clusters of and galaxies.
|